
Driving success through
MOF innovation
LEARN MORE
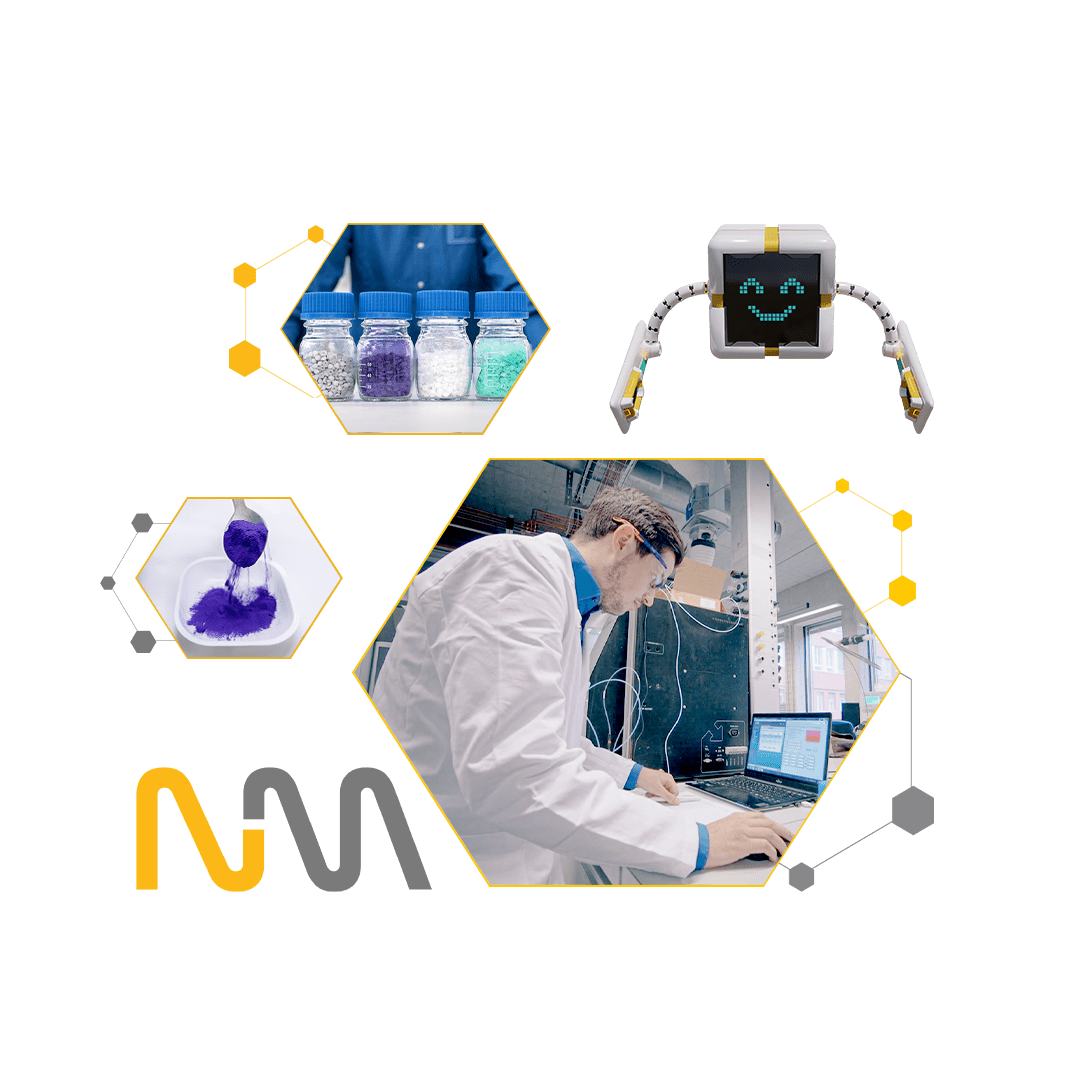
ABOUT US
Your strategic partner for innovative
MOF-based solutions
novoMOF focuses on the development, the production and the commercialization of metal-organic frameworks (MOFs). These highly porous adsorbents offer competitive solutions to global problems such as carbon capture, water scarcity, and food waste.
OUR TECHNOLOGY
A core focus of novoMOF is Carbon Capture
We’re pioneering a revolutionary solution to fight CO₂ emissions using Metal-Organic Frameworks, or MOFs. Our materials are a game-changer for both the climate and businesses.
Join us in creating a greener, more sustainable future. Reach out to novoMOF today to empower your business success with CO₂ capture.
Solution Process
1
SELECTION
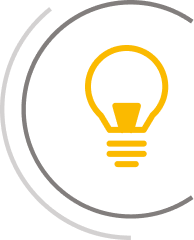
Your business challenges drive us in the identification of the right MOFs.
2
SYNTHESIS
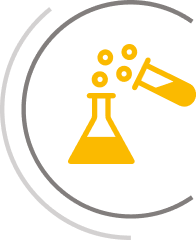
You receive synthesized MOFs at the highest quality.
You can test them for your application.
3
OPTIMIZATION
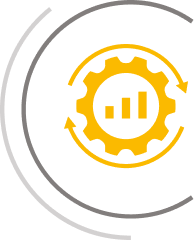
You benefit from support in optimizing the performance of your process.
4
PRODUCTION
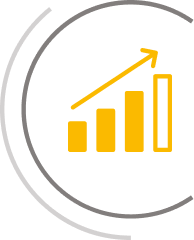
You leverage your commercial application with us, your trusted supply partner.
We scale production according to your needs from grams to tons.
WHY US
VALUE-FOCUSED
HIGH-QUALITY
EXPERTISE
SCALABLE
INNOVATIVE
Network
TESTIMONIALS
In our syngas conversion development efforts at Dow we have been investigating MOFs as catalyst precursors. In order to evaluate and compare our internal …
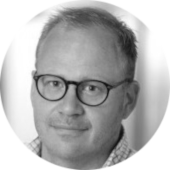
Matthijs Ruitenbeek
– Senior R&D Manager The Dow Chemical Company
As the chief scientific officer, I am always scouting for new technology platforms to improve our products at Silent-Power AG. I’ve met novoMOF at a conference in …
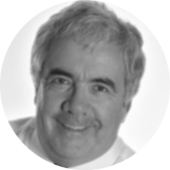
Dr. Reto Holzner
– Chief Scientific Officer econimo DRIVE
I had the opportunity to work with novoMOF AG on the scale up of one of our MOFs. Their production of a high-quality MOF at kilogram scale,…
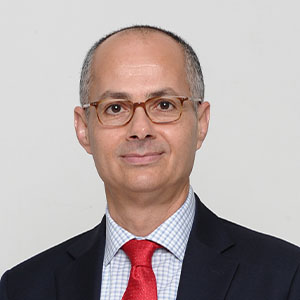
Dr. Omar M. Yaghi.
– Professor of Chemistry, UC Berkeley
novoMOF is a reliable partner for any organization seeking to scale up their metal-organic frameworks. As the principal investigator of our Atmospheric …
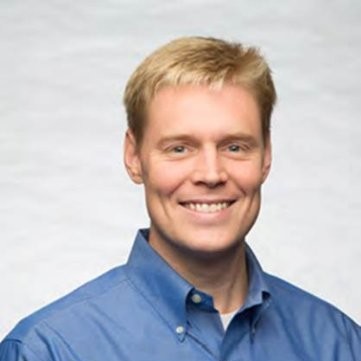
David R. Moore, Ph.D.
– GE Research Executive Manager, Carbon Capture Technology Leader
Recent Posts
novoMOF Blog Blog about Metal-Organic Frameworks (MOFs), their application and related industries.
- Towards carbon-neutralityon April 2, 2025 at 1:28 pm
Companies around the world are under growing pressure to reduce their carbon footprint and align with global sustainability goals. Governments and regulatory bodies are tightening emission standards and giving priority to businesses that demonstrate environmental responsibility. Achieving carbon neutrality has evolved from a corporate social responsibility initiative to a strategic necessity. This blog aims to help understand key aspects to achieve carbon neutrality, from carbon accounting to implementing reduction strategies to help companies navigate the transition toward a more sustainable future. CO₂ Neutral vs. CO₂ Negative When discussing carbon emissions, two key terms often come up: CO₂ neutral and CO₂ negative. While both indicate a commitment to reducing environmental impact, they represent different levels of action and ambition. CO₂ Neutral (Carbon Neutral): A company is considered CO₂ neutral when it balances the amount of carbon dioxide it emits with an equivalent amount removed from the atmosphere. This is typically achieved through emission reductions, increased efficiency, and carbon offsetting projects, such as reforestation or renewable energy investments. The goal is to achieve net-zero emissions, meaning the company does not contribute additional CO₂ to the atmosphere. CO₂ Negative (Carbon Negative): Going beyond neutrality, a CO₂ negative approach means that a company actively removes more carbon dioxide from the atmosphere than it emits. This can be done through advanced carbon capture technologies, large-scale reforestation, or direct air capture projects. Being CO₂ negative results in a net reduction of atmospheric CO₂, contributing significantly to reversing climate change. For companies, striving for CO₂ neutrality is often the first step in sustainability efforts, while CO₂ negativity represents a more ambitious goal for those aiming to have a positive environmental impact. Accounting for carbon emissions The first step towards carbon neutrality is to go through the identification process for accounting carbon emissions. The Greenhouse Gas (GHG) Protocol, the leading global standard for carbon accounting, categorizes emissions into three scopes based on their source and level of control by an organization. These scopes aim to help companies track and manage emissions systematically, allowing better reporting, regulatory compliance, and targeted mitigation strategies. Scope 1: Direct emissions. These emissions come from sources owned or controlled by the company. This scope includes production or process equipment to generate heat, steam, and electricity, such as boilers and furnaces, and mobile combustion equipment like vehicles. Scope 2: Indirect emissions. This scope includes emissions from the use of electricity generators not owned or controlled by the company (purchased electricity). Therefore, the emissions occur at the facility where electricity is generated. Scope 3: Other indirect emissions. This category includes emissions from downstream and upstream processes used by third parties along the value chain of a company. Scope 1 and 2 emissions are managed by the company through equipment selection and choosing their suppliers. Therefore, addressing scope 1 and 2 emissions is the starting point to tackle. Scope 3 emissions are harder to control and require collaboration with partners and suppliers. Implementing Reduction Strategies: Steel Manufacturing Example
- Unlocking the industrial potential of MOFson January 21, 2025 at 3:27 pm
Metal-Organic Frameworks are one of the most exciting materials of recent decades for the chemical industry. After the development of the first MOFs, one of the biggest challenges was to make them accessible for different processes. Their unparalleled surface areas, tunable porosity, and chemical versatility have enabled applications in gas storage, carbon capture, catalysis, and beyond. However, despite their potential, MOFs are not yet accessible on an industrial scale. Here, we aim to take the discussion further and explore what it truly means to make MOFs accessible at an industrial scale—and how we are achieving that vision. Industrial-Scale MOFs: What Does It Mean? The first step in producing MOFs is in the lab, on the gram scale. Industrial-scale production means producing MOFs at the kilotonne (kt) or megatonne (MT) level. The scale-up process does not just involve increasing the size of a reactor, but also requires considering critical factors such as: Raw material sustainability Production efficiency and robustness Product quality and performance retention Ease of downstream processing Achieving success in the scale-up process requires combining expertise and a profound understanding of MOF chemistry and engineering. How We Do It From years of experience, we conclude that scalability depends on one key factor: recipe stabilization. This means transforming fragile, lab-scale recipes into robust, reproducible processes. The stabilization process addresses every aspect of production including: Raw Materials and MOF Compositions: Raw materials are prioritized based on sustainability, ensuring a low environmental footprint from the start. They are also readily available, eliminating bottlenecks in the supply chain. For more on how MOFs contribute to the circular economy and address sustainability challenges, see our blog: Circular Economy - Tackling Challenges with Novel Materials. Solvents for Reaction, Work-Up, and Shaping: Traditional MOF recipes rely on toxic and expensive organic solvents like dimethylformamide (DMF). Organic solvents are avoided wherever possible, replacing them with greener alternatives. This shift improves safety, reduces costs, and aligns with global sustainability goals. Reaction Conditions: Key factors like pressure, temperature, additives, and reaction times are optimized to ensure: Reproducibility: Consistent results across batches. Efficiency: High yields with minimal waste. Scalability: The ability to use conventional manufacturing equipment at atmospheric pressure and low temperatures. Product Quality: Maintaining—and even improving—MOF performance at scale requires fine-tuning the synthetic procedure. For instance, adjustments in crystal size, porosity, or defect density can significantly impact gas adsorption, catalytic activity, or mechanical stability. For more insights, see our blog about How We Measure and Ensure the High Quality of Our MOFs. Product Rheology: Once produced, the MOF powder must be easily processed into its final form—whether as granules, coatings, or nanofibers. Our approach ensures that the as-synthesized material can transition seamlessly into application-specific formats without compromising quality or functionality. Learn more about how particle size affects MOF performance and processing in our blog: Particle Size of Metal-Organic Frameworks. Example of improved product rheology (left: big agglomerates make downstream processing more challenging; right: novoMOF's methods yield in MOFs in fine powder, comparable to baking flour, that facilitates further processing) A Recipe for Industrial Success
- Oxygen Production with MOFson October 11, 2024 at 3:48 pm
The global oxygen production market is a vital sector supporting medical, industrial, and environmental applications, with its significance growing steadily. Valued between USD 30 billion and USD 50 billion annually in 2023, the market is poised for substantial expansion driven by increasing demands from healthcare and industrial sectors, technological advancements, and heightened environmental initiatives. In the healthcare arena, oxygen is indispensable for treating respiratory conditions such as COPD, pneumonia, and during surgical procedures. The COVID-19 pandemic underscored the critical importance of robust oxygen supply chains, leading to significant investments in oxygen production technologies and infrastructure. This surge in demand extends to both hospitals and home healthcare settings, with emerging markets in Asia-Pacific and Africa exhibiting considerable growth potential. The pandemic also highlighted the need for scalable and low-cost oxygen purification solutions, especially in regions with limited infrastructure, emphasizing the market’s essential role in global health. Industrial applications of oxygen are equally crucial, particularly in steel manufacturing, chemical production, and wastewater treatment. The steel industry relies heavily on oxygen in blast furnaces to enhance efficiency and reduce energy consumption. Additionally, the global shift towards cleaner energy and sustainable industrial practices is boosting the demand for oxygen in environmental applications such as air and water purification. These trends reflect the broader move towards more sustainable and energy-efficient industrial processes, further driving the need for reliable oxygen production. Technological advancements are a key driver of market growth. Innovations in air separation units (ASUs) and pressure swing adsorption (PSA) systems have significantly improved the efficiency and cost-effectiveness of oxygen production. Moreover, the increasing focus on reducing carbon footprints has led to the development of green technologies for oxygen generation, enhancing the sustainability of the production process. These advancements not only lower operational costs but also expand the applicability of oxygen across various sectors. Looking ahead, the oxygen production market is expected to continue its upward trajectory as demand expands across multiple industries. Companies that invest in research and development and expand their production capacities are well-positioned to capitalize on this growth. The ongoing evolution of oxygen production technologies and the critical need for a reliable oxygen supply underscore the market’s pivotal role in supporting global industry and healthcare, ensuring its continued expansion in the coming years. The different separation technologies The production of oxygen-enriched gas streams is essential for various industrial and medical applications, relying on technologies that are often energy and infrastructure-intensive. The main methods include cryogenic distillation, pressure swing adsorption (PSA), vacuum pressure swing adsorption (VPSA), and membrane separation, each with distinct advantages and limitations. Cryogenic distillation is the most established technology, especially for large-scale industrial applications. It involves cooling air to cryogenic temperatures (-183°C) to liquefy its components. Oxygen is then separated based on its boiling point, allowing for the production of very high-purity oxygen. This method is highly effective and ideal for sectors requiring large volumes of pure oxygen, such as steelmaking, chemical manufacturing, and major healthcare facilities. However, the process is energy-intensive and requires significant infrastructure. Pressure swing adsorption (PSA) is a non-cryogenic method favored for its flexibility, particularly in smaller-scale operations. PSA systems use adsorbent materials, like zeolites, to selectively adsorb nitrogen from compressed air, allowing oxygen to pass through. While PSA is suitable for on-site oxygen generation in hospitals and industries requiring moderate oxygen purity, it is less efficient than cryogenic methods. Vacuum pressure swing adsorption (VPSA) is an advanced version of PSA, designed to improve efficiency and reduce operational costs. VPSA uses a vacuum to desorb nitrogen from the adsorbent materials more effectively, speeding up regeneration and lowering energy consumption. This method is particularly useful in applications needing large oxygen volumes at lower costs, such as wastewater treatment and glass manufacturing. Membrane separation is another non-cryogenic method, using selective membranes to separate oxygen from other gases. It is less energy-intensive and best suited for applications requiring lower oxygen purity, such as combustion enhancement and inerting. These technologies cater to diverse industrial needs, offering scalable, efficient, and cost-effective solutions for oxygen production, allowing businesses to choose the most suitable method based on their specific requirements. The separation process with MOF technology Over the past decade, significant efforts have been made to develop metal-organic frameworks (MOFs) as selective sorbents for oxygen capture, storage, and release. Two primary strategies have emerged, each involving different adsorption mechanisms. The first strategy involves MOFs that adsorb oxygen through a redox mechanism, where O₂ binds to open metal sites or bridging ligands. However, this approach is challenging because the strong binding of oxygen often makes its release difficult. The second strategy focuses on size-selective MOFs that preferentially adsorb oxygen over nitrogen. This approach also presents challenges due to the minimal size difference between O₂ and N₂ molecules. Recent studies have identified key requirements for MOFs suitable for air separation, including reversible sorption without structural collapse and a composition that allows for large-scale, cost-effective O₂ production. Additionally, it has been demonstrated that metal substitution within specific MOF families can fine-tune the selectivity and kinetics of the system, enhancing the O₂/N₂ separation performance. This improved performance is attributed to molecular sieving, which leverages the small size difference between the kinetic diameters of oxygen and nitrogen. Pore-size engineering in MOFs has thus been highlighted as a powerful tool for designing new materials for efficient air separation. Conclusion